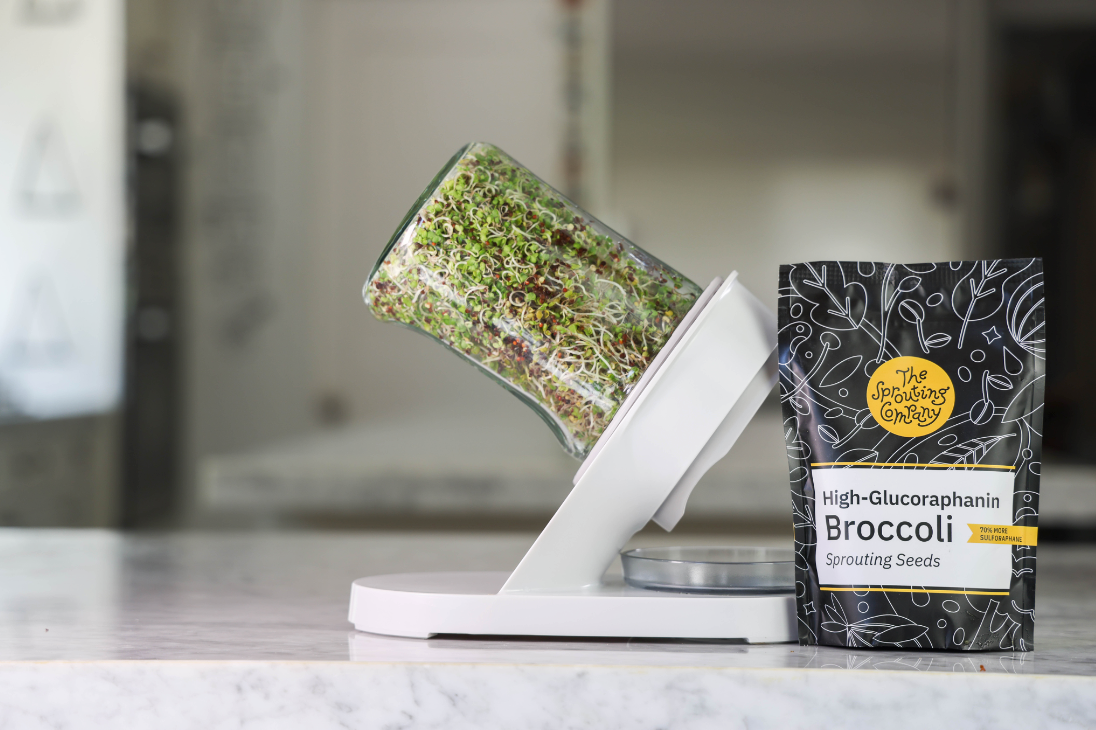
Boost Your Workout Recovery with Broccoli Sprouts
Share
Introduction
Ever wish you had a secret weapon to enhance your workouts and speed up recovery? Turns out, the answer might be sitting right in your fridge—broccoli sprouts! These tiny greens pack a punch when it comes to supporting exercise-induced improvements. A recent study titled "Glucosinolate-rich broccoli sprouts protect against oxidative stress and improve adaptations to intense exercise training" by Flockhart et al. (2023) dives into how these sprouts can be a game-changer for anyone looking to get the most out of their fitness routine.
Why Broccoli Sprouts?
Broccoli sprouts are more than just a trendy garnish; they’re a nutritional powerhouse loaded with compounds that can help your body perform better and recover faster. Flockhart et al. (2023) reveal that these sprouts are especially high in sulforaphane, a potent antioxidant known for its ability to reduce inflammation and support overall health. But what does this mean for your workouts?
The Study: How Broccoli Sprouts Enhance Exercise Benefits
The study by Flockhart et al. (2023) explored the effects of broccoli sprouts on individuals undergoing regular exercise. Here’s what they found:
Reduced Muscle Inflammation
One of the standout findings was that participants who included broccoli sprouts in their diet experienced less muscle inflammation after exercise. This means less soreness and a quicker recovery, allowing you to get back to your workouts faster.
Improved Antioxidant Defense
Exercise increases oxidative stress in the body, which can lead to muscle fatigue and slower recovery. The sulforaphane in broccoli sprouts helps boost your body’s natural antioxidant defenses, combating oxidative stress and helping your muscles recover more efficiently.
Enhanced Endurance
The study also noted improvements in endurance among participants who consumed these sprouts regularly. By reducing inflammation and oxidative stress, broccoli sprouts help your body perform better during endurance activities like running, cycling, or any other high-intensity workout.
Support for Overall Health
Beyond just exercise, the compounds in broccoli sprouts support overall health, including better heart function and improved immune response. It’s a simple addition to your diet that offers benefits far beyond the gym.
How to Incorporate Broccoli Sprouts into Your Routine
Ready to give your workouts a boost? Here are some easy ways to add broccoli sprouts to your diet:
- Smoothies: Toss a handful of broccoli sprouts into your post-workout smoothie. They blend right in and give you an extra dose of recovery power.
- Salads: Add these sprouts to your salads for a crunchy, nutrient-packed topping that supports your exercise goals.
- Wraps and Sandwiches: Layer them onto sandwiches or wraps for a fresh, peppery kick that enhances both flavor and nutrition.
- Stir-Fries: Broccoli sprouts can also be added to stir-fries at the end of cooking for a light, crunchy addition that’s packed with benefits.
The best part? You can easily grow broccoli sprouts at home. The Sprouting Company offers simple kits that let you grow fresh, organic sprouts in just a few days. Not only do you get all the benefits of these potent little greens, but you also get the satisfaction of growing them yourself.
With their unique ability to reduce inflammation, enhance antioxidant defense, and improve endurance, broccoli sprouts are a no-brainer for anyone looking to optimize their fitness routine. Start incorporating them into your meals today and feel the difference they can make in your workouts and recovery.
Want to Learn More? Dive Into the Science
For a deeper dive into how broccoli sprouts can support your exercise routine, check out the full study written below, titled "Glucosinolate-rich broccoli sprouts protect against oxidative stress and improve adaptations to intense exercise training" by Flockhart et al. (2023). The research offers valuable insights into why these tiny sprouts should be a staple in your diet if you’re serious about your fitness goals.
Conclusion: Small Sprouts, Big Gains
Don’t underestimate the power of these tiny greens. Broccoli sprouts offer a simple, natural way to boost your workouts, speed up recovery, and support your overall health. Whether you’re an athlete or just getting started with fitness, these sprouts are an easy addition that can make a big difference. Start sprouting today and take your performance to the next level!
--
Full Study: Glucosinolate-rich broccoli sprouts protect against oxidative stress and improve adaptations to intense exercise training
By M. Flockhart, L.C. Nilsson, E.N. Tillqvist, F. Vinge, F. Millbert, J. Lännerström, P.H. Nilsson, D. Samyn, W. Apró, M.L. Sundqvist, F.J. Larsen
Abstract
Oxidative stress plays a vital role in the adaptive responses to physical training. However, excessive oxidative stress can precipitate cellular damage, necessitating protective mechanisms to mitigate this effect. Glucosinolates, found predominantly in cruciferous vegetables, can be converted into isothiocyanates, known for their antioxidative properties. These compounds activate crucial antioxidant defense pathways and support mitochondrial function and protein integrity under oxidative stress, in both Nrf2-dependent and independent manners. We administered glucosinolate-rich broccoli sprouts (GRS) in a randomized double-blinded cross-over fashion to 9 healthy subjects in combination with daily intense exercise training for 7 days. We found that exercise in combination with GRS significantly decreased the levels of carbonylated proteins in skeletal muscle and the release of myeloperoxidase into blood. Moreover, it lowered lactate accumulation during submaximal exercise and attenuated the severe nocturnal hypoglycemic episodes seen during the placebo condition. Furthermore, GRS in combination with exercise improved physical performance, which was unchanged in the placebo condition.
Introduction
Short periods of intense exercise training have been shown to induce substantial metabolic and circulatory adaptations that can improve health and physical performance. At the same time, exercise training imposes a significant organismal challenge with increased oxidative and energetic stress that needs to be managed through homeostatic mechanisms. One such mechanism is the transcription factor nuclear factor erythroid 2-related factor 2 (Nrf2) which activates critical endogenous antioxidant defense pathways and maintains mitochondrial function and protein integrity during oxidative stress. Also, Nrf2 is crucial in coordinating the adaptive response to exercise training.
Lack of Nrf2 has been shown to negatively affect skeletal muscle function after exercise in aged mice and it also causes a faster decline in physical performance and muscle mass with age. Acute exercise seems to increase mRNA of Nrf2 in both mice and humans as a response to oxidative stress. Although moderate exercise activates Nrf2, periods of excessive exercise have been shown to decrease Nrf2 expression and impair mitochondrial respiration with a simultaneous loss of metabolic adaptations and induce disturbances in glucose control.
Extensive research has demonstrated that the administration of direct antioxidants, such as vitamin C or E, during periods of exercise training may hamper the adaptive responses associated with training under certain circumstances. These findings suggest that exercise-induced oxidative stress could exert advantageous signaling effects, contributing to the beneficial adaptations observed in response to exercise. Similarly, a cellular environment subtly skewed towards pro-oxidant conditions may be associated with improved adaptations to exercise training.
Expanding on the interplay between oxidative stress and exercise adaptations, isothiocyanates deserve attention. Isothiocyanates are a group of chemicals derived from glucosinolates found in plants in the Brassica genus that includes broccoli, kale, cauliflower, rocket, etc. Upon chewing or mechanical processing, glucosinolates become hydrolyzed to isothiocyanates by the enzyme myrosinase. Although more than 100 isothiocyanates have been identified to date and several have Nrf2-activating and other potent properties, the by far most studied isothiocyanate is sulforaphane. In addition to being potential Nrf2-activators, isothiocyanates also have Nrf2-independent properties, including inhibition of mitochondrial fission and the mTOR pathway.
Methods
Preparation of Sprouts
Broccoli raab sprouts and alfalfa sprouts (placebo) were grown in a commercial sprout growing facility (Munka-Grodden AB, Sweden). The sprouts were harvested on day 5 after initial soaking. After repeated washing, sprouts were homogenized in water with a ratio of 75 g of sprouts to 180 mL of water. The sprout drinks were then immediately frozen to −80°C. Upon consumption, the drinks were quickly thawed, and 50 mL apple juice concentrate was added for improved taste and masking, together with 0.75 g brown mustard seed powder containing myrosinase to facilitate the conversion of glucosinolates to isothiocyanates. Measurement of glucosinolates was conducted according to Mawlong et al. with slight modifications. The broccoli sprouts contained 1.145 ± 0.035 mmol of total glucosinolates per 75-g fresh weight and the glucosinolate content in the alfalfa sprouts was 0.014 ± 0.001 mmol per 75-g fresh weight. The energy content of one dose of sprout drink including the apple juice was 90 kcal for the broccoli sprout drink and 84 kcal for the alfalfa sprout drink.
Sprout Drink Supplementation
The study design was a randomized, double-blinded, cross-over study with a 1-month wash-out between the different treatments. Subjects consumed 2 bottles of sprout juice per day, one in the morning and one in the evening. The bottles contained 75 g of sprouts each, and the supplementation period continued for 9 days. The last GRS or PLA drink was consumed 78 ± 4 minutes before the post-tests started. During the wash-out period, the subjects were instructed to follow their habitual diet and exercise pattern.
Standardization of Diet
Subjects maintained their usual diet throughout the training week, specifically excluding glucosinolate-rich foods such as cabbage, kale, broccoli, and cauliflower. Participants were asked to keep a detailed food record, noting everything they consumed. This helped monitor adherence to the dietary instructions and assess any potential influence of uncontrolled dietary variations. On the evening prior to pre- and post-tests, subjects consumed a standardized meal of 785 kcal (35 g fat, 98 g carbohydrates, 15 g proteins) provided by the research team. Subsequently, they fasted overnight, establishing a consistent pre-test nutritional state across all participants.
Training Protocol
All nine subjects completed the seven training sessions in the intervention period. The sessions were self-paced, and the subjects were instructed to produce the highest mean power output during the part of the sessions that included repetitions of 4–8 minutes. Each 30-second sprint was performed in an all-out mode. The average power output during training was 204 ± 27 W during PLA and 204 ± 29 W during GRS. Average blood lactate at the end of the sessions was 11.7 ± 2.3 mM in PLA and 11.0 ± 2.0 mM in GRS (p = 0.28). The average heart rate during the training sessions was 168 ± 10 bpm in PLA and 169 ± 8 bpm in GRS (p = 0.58).
Pre- and Post-Test
Pre- and post-training intervention, physiological characteristics during cycling were assessed using an SRM ergometer. Submaximal work rate response was evaluated with 5-minute intervals at fixed work rate and cadence, each separated by a minute of rest. Capillary blood lactate and glucose levels were measured and analyzed with a Biosen C-Line Clinic. The initial work rate was set to 80–120 W, then increased by 15–30 W per stage until blood lactate accumulation >4 mM was observed. After a brief rest, an incremental maximal exercise test was conducted to establish VO2max. The test began at the previously achieved work rate, with 15–20 W increases per minute until fatigue. VO2max was determined from the highest average 30 and 45-second periods, respectively. Gas exchange was continuously measured using an Oxycon Pro device, heart rate was recorded, and perceived exertion rated at each stage via the Borg RPE-scale. All equipment was calibrated as per manufacturers’ instructions.
Muscle Biopsies
Biopsies were collected from fasting subjects early in the morning after a 12-hour rest period following the last HIIT session. Pre and post biopsies were taken from alternating legs in the vastus lateralis region using a randomized order among subjects. Local anesthesia was applied at the biopsy site before extracting roughly 100 mg of wet tissue using a needle biopsy. The samples were cleaned of visible fat, connective tissue, and blood, then divided into two parts: ~50 mg for respirometric analysis in ice-cold ISO medium, and the other part was flash-frozen in liquid nitrogen for later analysis.
Mitochondrial Respiration
Mitochondria were extracted from fresh muscle in isolation medium following Gnaiger and Kuznetsov’s method with modifications by Tonkonogi and Sahlin, and Larsen et al. The muscle was chilled, homogenized, then treated with bacterial protease, followed by centrifugation to separate and re-suspend pellets. Respiration of isolated mitochondria was gauged using a two-channel respirometer in respiration medium MIR05 at 37°C, following manufacturers’ calibration instructions. Respiration was assessed across various states using different combinations of substrates including octanoyl carnitine, malate, pyruvate, ADP, glutamate, rotenone, and succinate.
Glycogen
Muscle glycogen was evaluated in roughly 2 mg of freeze-dried, dissected muscle following a slightly modified version of a previously described homogenization protocol. In brief, all samples were first heated in 1 M KOH (70°C for 20 minutes) and then incubated in NaAc + Hac and NaAc + amyloglucosidase (40°C for 2 hours). After a second incubation with reagents (20 minutes), the absorbance was read at 340 nm using a Beckman Coulter DU800 spectrophotometer.
Assessment of MDA and Carbonyl Content
Carbonyl content was determined in approximately 1 mg of freeze-dried muscle samples, dissolved in 1 M KOH and heated at 70°C using the Abcam Protein Carbonyl Content Assay Kit. After DNPH and TCA were added to the samples, centrifugation was performed, and the pellet was dissolved in acetone. The mixture was blended and centrifuged again. The pellet was then dissolved in guanidine solution and added to a 96-well microplate for absorbance reading at 360 nm using a TECAN Infinite 200 Pro spectrophotometer. Protein content was then determined using Thermo Scientific Pierce Protein Assay Kit and measured at 660 nm.
Malondialdehyde (MDA) was evaluated using the Sigma Aldrich Lipid Peroxidation Assay Kit. The assay involves MDA’s reaction with thiobarbituric acid (TBA) to form a colorimetric product indicative of MDA content. Homogenate containing about 1 mg freeze-dried muscle was added to MDA standard solution and BHT. After TBA solution was added and incubated at 95°C for an hour, absorbance was measured at 532 nm in a Beckman Coulter DU800 Spectrophotometer.
Myeloperoxidase
Myeloperoxidase was measured in EDTA-plasma using the myeloperoxidase DuoSet ELISA kit according to the manufacturer’s instructions. All samples were diluted 1:100 in 1% BSA in PBS.
Continuous Glucose Monitoring
The participants were outfitted with a FreeStyle Libre™ continuous glucose monitor (CGM) sensor. This device was strategically positioned on the deltoids' posterior surface, adhering to the manufacturer’s detailed guidelines. To ensure data integrity and minimize gaps in the data collection, participants received clear instructions to use the dedicated CGM reader for sensor scans at regular intervals, approximately every 8 hours.
Insulin
The quantification of plasma insulin levels was carried out utilizing an ELISA kit (Invitrogen, KAQ1251), following the guidelines provided by the manufacturer.
Western Blot
Freeze-dried muscle was used for immunoblotting. The homogenization protocol has previously been described in detail as well as the protocol and material for immunoblotting. The Nrf2 antibody was purchased from Cell Signaling Technology. The proteins from each subject were loaded on the same gels and in series, but in randomized order for treatment (PLA and GRS). The whole lanes on the Memcode were used as a loading control. Molecular Imager ChemiDoc XRS system with Image Lab software was used for visualization and quantification.
Statistics
A two-way analysis of variance (ANOVA) was used to assess the effects of a week of exercise combined with either GRS supplementation or PLA on various outcome measures. The two-way ANOVA allowed us to investigate both the main effects of the intervention (exercise) as well as the interaction effects between the different treatments. To control for multiple comparisons, we employed post-hoc tests using the Holm-Sidak method. We also examined the main effects of the intervention and time on a priori specified parameters (Nrf2 expression and performance) to provide a comprehensive understanding of the data. In cases where pairwise comparisons were necessary to compare different groups, we utilized independent and paired sample t-tests, as appropriate. One subject failed to complete the maximal exercise pre-test during the placebo condition due to a vasovagal reaction and was excluded from the analysis in certain figures. Values are mean ± S.E.M. p < 0.05 was considered significant.
Results
Skeletal Muscle Nrf2 Protein is Modulated after GRS and Exercise and Protects Against Oxidative Stress
We first wanted to understand if the intense exercise with GRS or PLA had increased the protein abundance of Nrf2 and activated the antioxidative defense system. We therefore measured Nrf2 in skeletal muscle homogenates using western blot technique. We found a near-significant main effect of training (p = 0.09) and a post-hoc test revealed that Nrf2 was significantly upregulated only in the GRS condition after exercise training (p = 0.03, pre compared to post) but not in the PLA condition (p = 0.83, pre compared to post, Sidak’s post-hoc test). Nrf2 activation is associated with the induction of antioxidative genes, which should protect against exercise-induced oxidative stress. To assess whether GRS treatment also affected markers of oxidative stress, we measured carbonyl content and malondialdehyde levels in the skeletal muscle homogenates. We found a significant interaction effect on carbonyl content between the GRS and PLA conditions. From pre to post-exercise, carbonyl content increased from 0.64 ± 0.06 to 0.71 ± 0.05 in the PLA condition and decreased from 0.75 ± 0.06 to 0.65 ± 0.05 in GRS (p = 0.03 by interaction). However, malondialdehyde levels were not changed by either exercise or treatment (from 237 ± 20 to 270 ± 34 in PLA and from 200 ± 17 to 234 ± 17 in GRS). Thereafter, we measured myeloperoxidase concentrations in plasma samples to investigate if the altered redox status of the muscle cells was also reflected in the blood. Indeed, we found that myeloperoxidase increased from 29.6 ± 2.6 to 32.4 ± 2.4 in the PLA condition and decreased from 32.5 ± 2.3 to 27.9 ± 2.3 ng/mL in the GRS condition (p = 0.002 by interaction). Together, these results indicate an increased antioxidative defense and a marked reduction in oxidative stress after GRS-treatment compared to PLA despite a more modest upregulation of Nrf2. Considering that the functional activation of Nrf2 is driven by its translocation into the nucleus, the modest elevation of Nrf2 observed here in whole muscle homogenates could indeed correspond to a substantial increase at the nuclear compartment, thereby having a more pronounced effect on antioxidant gene expression.
GRS Decreases Lactate Accumulation During Submaximal Work and Improves Maximal Performance
In animal studies, it has been demonstrated that the activation of Nrf2 enhances exercise performance. A critical component of physical performance improvement is the diminished accumulation of lactate during submaximal work, which indicates a primary adaptive response.
To investigate this further, we assessed capillary lactate levels during submaximal exercise. Notably, we observed a significant interaction effect where the lactate concentrations were markedly lower following the administration of GRS, as compared to PLA, under submaximal workload conditions. During submaximal work, the average lactate concentration increased in PLA from pre 2.69 ± 0.24 to post 3.17 ± 0.35 mM and decreased in GRS from pre 3.06 ± 0.24 to post 2.66 ± 0.20 mM (p = 0.003 by interaction). The work rate at an absolute lactate concentration was improved by 15–20 W or ~15%. During an incremental exercise test to exhaustion, maximal oxygen consumption (VO2max) was not significantly affected (3.12–3.14 L min−1 in PLA and 3.13–3.22 L min−1 in GRS, main effect p = 0.10, interaction effect p = 0.19). However, time to exhaustion during the maximal test was significantly improved in the GRS condition (from 401 ± 19 to 406 ± 22 s in PLA and 380 ± 22 to 426 ± 21 s in GRS, p = 0.02 by interaction). A well-known negative effect of intense periods of training is a reduction in maximum heart rate. Here we found maximum heart rate significantly decreased from 187 ± 3 to 184 ± 3 bpm in PLA but unchanged from 185 ± 3 to 185 ± 3 bpm in the GRS, p = 0.03 by interaction.
A change in lactate concentrations during submaximal exercise could indicate a change in substrate preference. Therefore, we measured oxygen consumption (VO2) and carbon dioxide emission (VCO2) during submaximal work rate where the respiratory exchange ratio (RER) (VCO2/VO2) reflects substrate utilization. We found no main effects of the intervention but there was a tendency towards an interaction effect (p = 0.06) for a difference in RER at moderate work rates from 0.968 ± 0.01 to 0.982 ± 0.01 in PLA and from 0.973 ± 0.01 to 0.971 ± 0.01 possibly indicating a shift towards higher reliance on lipid oxidation after GRS compared to PLA.
Mitochondrial Respiration and Content are Unchanged After GRS and PLA
Increases in mitochondrial capacity and function are expected outcomes of endurance exercise training. At a given submaximal work rate, a higher mitochondrial capacity should divert more pyruvate into mitochondrial oxidation instead of a reduction to lactate by lactate dehydrogenase. To understand if the lower lactate concentrations during exercise after GRS is due to an increase in mitochondrial content, we assessed the activity of citrate synthase in the muscle tissue, but found it was unchanged in both conditions (from 6.5 ± 2.4 to 6.9 ± 4.6 in PLA and 6.6 ± 2.3 to 6.9 ± 3.0 μmol min−1 g−1 dw in GRS). We also measured mitochondrial function by High-Resolution Respirometry in isolated mitochondria but found no significant main or interaction effect by the intervention. Therefore, other mechanisms than an increased mitochondrial capacity must be responsible for the reduction in lactate.
GRS Markedly Attenuates Nocturnal Hypoglycemia During Periods with Intense Exercise Training
Intense exercise training periods seem to correlate with a higher incidence of both hypo- and hyperglycemic episodes in elite athletes. Sulforaphane has been demonstrated to normalize exaggerated hepatic glucose production and improve HbA1c in specific diabetic patient subgroups. We aimed to corroborate these findings in our current study population, investigating whether GRS-rich sprouts could improve blood glucose control. Interstitial glucose was continuously monitored throughout the seven-day-long training period, revealing that time spent with glucose below 4 mM averaged 5 hours 21 minutes ± 67 minutes per day in the PLA group. In contrast, it decreased to 2 hours 16 minutes ± 51 minutes daily in the GRS group (p = 0.002). Additionally, the mean blood glucose was significantly lower (4.67 ± 0.11 mM in PLA versus 5.02 ± 0.10 mM in GRS, p < 0.0001). Nonetheless, the time with blood glucose exceeding 8 mM was similar in both groups (10 ± 6 minutes in PLA and 16 ± 4 minutes in GRS per 24 hours). Despite the substantial changes in CGM readings, the fasting insulin levels at the post-tests were unchanged between conditions (7.5 ± 1.4 mU L−1 in PLA, and 7.4 ± 1.4 mU L−1 in GRS, p = 0.92, t-test). The CGM readings represent weekly average readings across all subjects. However, given that meals and training sessions took place at slightly different times each day throughout the week, this approach might overlook subtle variations in the data. We therefore looked more specifically at the glucose response at the time around each exercise session. We discovered a significant interaction effect that pointed to distinct glucose responses under the GRS and PLA conditions. During exercise, the glucose level in the GRS group was slightly elevated but remained stable. In contrast, the PLA group started with a lower glucose level, peaked to match the GRS group towards the end of the exercise, but then dipped to a lower level again. Statistically, these variations were significant; treatment effect p = 0.04 and interaction effect p = 0.001. Given the potential differences between glucose levels measured in the interstitial fluid and in the blood, we sought to validate our findings using capillary blood measurements taken during the post-test. Although these tests were performed 24–72 hours after the last training session, we observed a significant treatment effect, characterized by marginally elevated blood glucose levels during submaximal exercise and following the maximal exercise test (p = 0.02 by treatment effect). This in-depth analysis underscores the differential impact of the GRS and PLA treatments on glucose dynamics during exercise.
Discussion
contHere we show that incorporating supplementation with glucosinolate-rich (GRS) broccoli sprouts into a 7-day intense training regimen in a cohort of healthy subjects not only mitigates several markers of oxidative stress and improves the blood glucose profile but also enhances the exercise-induced adaptations observed in the participants.
We also observed a reduction in carbonylated proteins within muscle tissue and myeloperoxidase levels in the blood following one week of GRS administration and training. This indicates that the overall adaptations to acute oxidative stress induced by exercise and GRS were towards diminished oxidative stress and improved physical performance. In contrast, oxidative stress increased during the placebo condition with blunted physiological adaptations.
Human exercise studies utilizing glucosinolate or isothiocyanate administration are sparse. However, in a recent study by Komine et al., it was demonstrated that a two-week regimen of sulforaphane supplementation prior to engaging in eccentric exercise effectively mitigated both delayed onset of muscle soreness and markers of oxidative stress in human subjects. Notably, this was accompanied by an increase in NQO1 mRNA expression in blood samples. Similarly, Oh et al. discovered that sulforaphane injections in wild-type mice significantly bolstered running endurance. This improvement was attributed to the upregulation of Nrf2 signaling and the mitigation of oxidative stress-induced muscle damage during exhaustive exercise, thereby highlighting the potential role of sulforaphane in augmenting exercise performance.
Although the beneficial effects of moderate exercise are well-known, periods with excessive exercise have been shown to have negative effects on both muscle and metabolic function. In a previous investigation, we demonstrated the emergence of reduced glucose tolerance and loss of mitochondrial function following a three-week progressively intensified training period. Building upon these findings, the current study employed a comparable training protocol, specifically focusing on the final week of the three-week period. Surprisingly, despite the implementation of this rigorous training week, we did not observe a reduction in mitochondrial function, irrespective of the administered supplementation indicating that the training load was well accepted by our subjects.
The markedly reduced hypoglycemia in the present study suggests that factors beyond carbohydrate intake and energy balance, such as oxidative stress, might play a pivotal role in glucose regulation. This highlights the complexity of metabolic responses to strenuous exercise and indicates the need for further investigations into the relative contributions of energy balance and oxidative stress to glucose homeostasis in athletes.
An unexpected finding in this study was that blood lactate concentrations during submaximal exercise were lower after GRS compared to PLA. The lower lactate accumulation often seen after a period of training is often attributed to either an increased oxygen delivery or improved mitochondrial capacity. We did not find any significant differences in either VO2max or mitochondrial function or capacity, indicating that other, unknown mechanisms were at play.
The observed decrease in lactate levels may have broader implications beyond the scope of exercise physiology. Isothiocyanates, for instance, are postulated to exhibit chemopreventive properties and have demonstrated inhibitory effects on cancer development across various cellular and animal models. Singh et al. recently demonstrated that sulforaphane effectively inhibited cancer cell proliferation by suppressing glycolysis and consequently diminishing the activity of several glycolytic enzymes. This led to a consequential decrease in lactate accumulation. However, when resting plasma lactate levels in prostate cancer patients were analyzed post-administration of broccoli sprout extract, no significant reductions in lactate levels were detected.
The observation of increased interstitial glucose throughout the day, as measured by continuous glucose monitors, coupled with decreased lactate levels is intriguing. One plausible explanation for this pattern could be enhanced gluconeogenesis, leading to increased conversion of lactate to glucose.
Alternatively, the observation might be attributed to an upregulation in the reverse reaction of lactate dehydrogenase, where lactate is converted back to pyruvate. This would subsequently make more pyruvate available for further oxidation in mitochondrial metabolism. Our data, which indicated a trend towards a lower respiratory exchange ratio (RER) during submaximal exercise and a less pronounced increase in glycogen following GRS administration, supports this hypothesis. These findings suggest a decreased reliance on glycogen as a metabolic substrate following the consumption of GRSs.
In summary, supplementation with GRS in combination with intense exercise training seems to ameliorate oxidative stress and improve the physiological adaptations to the training program with lower lactate concentrations during exercise and attenuated time spent in the hypoglycemic range. The mechanism behind these novel findings is subject to future investigations.
Funding
This study was funded by grants from Ekhagastiftelsen, the Swedish Research Council for Sport Science, and Sydgrönt Ekonomisk Förening.
Declaration of Generative AI and AI-Assisted Technologies in the Writing Process
During the preparation of this work, the authors used MS Word text prediction and Open AI services in order to check grammar and language. After using these services, the authors reviewed and edited the content as needed and take full responsibility for the content of the publication.
Declaration of Competing Interest
This study was partially sponsored by Sydgrönt, an economic association for vegetable growers. The sprouts used in the study were generously provided by Munka-Grodden AB, a company specializing in the commercial cultivation of sprouts. These organizations did not play any role in the conduct, analysis, or interpretation of the study’s data. Authors FJL and MLS hold a patent related to the use of isothiocyanates for enhancing adaptations to exercise training. In addition, they are co-founders and shareholders in a commercially operational entity, which aims to market and sell products designed to augment athletic performance. No other authors have any conflicts of interest to declare.
Data Availability
Data will be made available on request.
References
Margonis K, et al. Oxidative stress biomarkers responses to physical overtraining: implications for diagnosis. Free Radic Biol Med. 2007;43(6):901–910.
Esteras N, Abramov AY. Nrf2 as a regulator of mitochondrial function: energy metabolism and beyond. Free Radic Biol Med. 2022;189:136–153.
Vargas-Mendoza N, et al. Antioxidant and adaptive response mediated by Nrf2 during physical exercise. Antioxidants. 2019;8(6).
Yan X, et al. Nrf2 contributes to the benefits of exercise interventions on age-related skeletal muscle disorder via regulating Drp1 stability and mitochondrial fission. Free Radic Biol Med. 2022;178:59–75.
Huang DD, et al. Nrf2 deficiency exacerbates frailty and sarcopenia by impairing skeletal muscle mitochondrial biogenesis and dynamics in an age-dependent manner. Exp Gerontol. 2019;119:61–73.
Flockhart M, et al. Excessive exercise training causes mitochondrial functional impairment and decreases glucose tolerance in healthy volunteers. Cell Metab. 2021;33(5):957–970.e6.
Merry TL, Ristow M. Do antioxidant supplements interfere with skeletal muscle adaptation to exercise training? J Physiol. 2016;594(18):5135–5147.
Margaritelis NV, et al. Adaptations to endurance training depend on exercise-induced oxidative stress: exploiting redox interindividual variability. Acta Physiol (Oxf). 2018;222(2).
O’Mealey GB, Berry WL, Plafker SM. Sulforaphane is a Nrf2-independent inhibitor of mitochondrial fission. Redox Biol. 2017;11:103–110.
Zhang Y, et al. The isothiocyanate sulforaphane inhibits mTOR in an NRF2-independent manner. Phytomedicine. 2021;86:153062.
Valgimigli L, Iori R. Antioxidant and pro-oxidant capacities of ITCs. Environ Mol Mutagen. 2009;50(3):222–237.
Fahey JW, Zhang Y, Talalay P. Broccoli sprouts: an exceptionally rich source of inducers of enzymes that protect against chemical carcinogens. Proc Natl Acad Sci USA. 1997;94(19):10367–10372.
Oh S, et al. Nuclear factor (erythroid-derived 2)-like 2 activation increases exercise endurance capacity via redox modulation in skeletal muscles. Sci Rep. 2017;7(1):12902.
Housley L, et al. Untargeted metabolomic screen reveals changes in human plasma metabolite profiles following consumption of fresh broccoli sprouts. Mol Nutr Food Res. 2018;62(19)
.
Wang L, et al. Nrf2 activation enhances muscular MCT1 expression and hypoxic exercise capacity. Med Sci Sports Exerc. 2020;52(8):1719–1728.
Yoshioka M, et al. Effect of SM-2470, a newly synthesized alpha 1-adrenoceptor antagonist, on sympathetic nerve activity in anesthetized rats. Arch Int Pharmacodyn Ther. 1988;293:162–174.
Komine S, et al. Effect of a sulforaphane supplement on muscle soreness and damage induced by eccentric exercise in young adults: a pilot study. Physiol Rep. 2021;9(24)
.
Sato K, et al. Oral chronic sulforaphane effects on heavy resistance exercise: implications for inflammatory and muscle damage parameters in young practitioners. Nutrition. 2021;90:111266.
Okunade OA, et al. Thermal and pressure stability of myrosinase enzymes from black mustard (Brassica nigra L. W.D.J. Koch. var. nigra), brown mustard (Brassica juncea L. Czern. var. juncea) and yellow mustard (Sinapsis alba L. subsp. maire) seeds. Food Chem. 2015;187:485–490.
Mawlong I, et al. A simple spectrophotometric method for estimating total glucosinolates in mustard de-oiled cake. Int J Food Prop. 2017;20(12):3274–3281.
Borg GA. Psychophysical bases of perceived exertion. Med Sci Sports Exerc. 1982;14(5):377–381.
Gnaiger E, Kuznetsov AV. Mitochondrial respiration at low levels of oxygen and cytochrome c. Biochem Soc Trans. 2002;30(2):252–258.
Tonkonogi M, Sahlin K. Rate of oxidative phosphorylation in isolated mitochondria from human skeletal muscle: effect of training status. Acta Physiol Scand. 1997;161(3):345–353.
Larsen FJ, et al. Mitochondrial oxygen affinity predicts basal metabolic rate in humans. FASEB J. 2011;25(8):2843–2852.
Leighton B, et al. Acute and chronic effects of strenuous exercise on glucose metabolism in isolated, incubated soleus muscle of exercise-trained rats. Acta Physiol Scand. 1989;136(2):177–184.
Samuelsson H, et al. Intake of branched-chain or essential amino acids attenuates the elevation in muscle levels of PGC-1α4 mRNA caused by resistance exercise. Am J Physiol Endocrinol Metab. 2016;311(1)
–E251.
Moberg M, et al. Activation of mTORC1 by leucine is potentiated by branched-chain amino acids and even more so by essential amino acids following resistance exercise. Am J Physiol Cell Physiol. 2016;310(11)
–C884.
Axelsson AS, et al. Sulforaphane reduces hepatic glucose production and improves glucose control in patients with type 2 diabetes. Sci Transl Med. 2017;9(394).
Na G, et al. Dietary isothiocyanates: novel insights into the potential for cancer prevention and therapy. Int J Mol Sci. 2023;24(3).
Singh KB, et al. Reversal of the Warburg phenomenon in chemoprevention of prostate cancer by sulforaphane. Carcinogenesis. 2019;40(12):1545–1556.
--
M. Flockhart, L.C. Nilsson, E.N. Tillqvist, F. Vinge, F. Millbert, J. Lännerström, P.H. Nilsson, D. Samyn, W. Apró, M.L. Sundqvist, F.J. Larsen (2023). Glucosinolate-rich broccoli sprouts protect against oxidative stress and improve adaptations to intense exercise training. Redox Biology, 64, 102838. https://doi.org/10.1016/j.redox.2023.102873